Fig. 4 -Area function (Top), and some waveforms obtained
from the simulation. $P_{Out}$ is the acoustic waveform radiated at the
lips, $h_t$ is the height of the lingual constriction at the upstream
(solid line) and the downstream (dashed line) sections. $U_m$ is the
volme velocity of the airflow at the mouth, and $h_g$ is the glottal
opening at the upstream (solid line) and downstream (dashed line) parts
of the vocal folds.
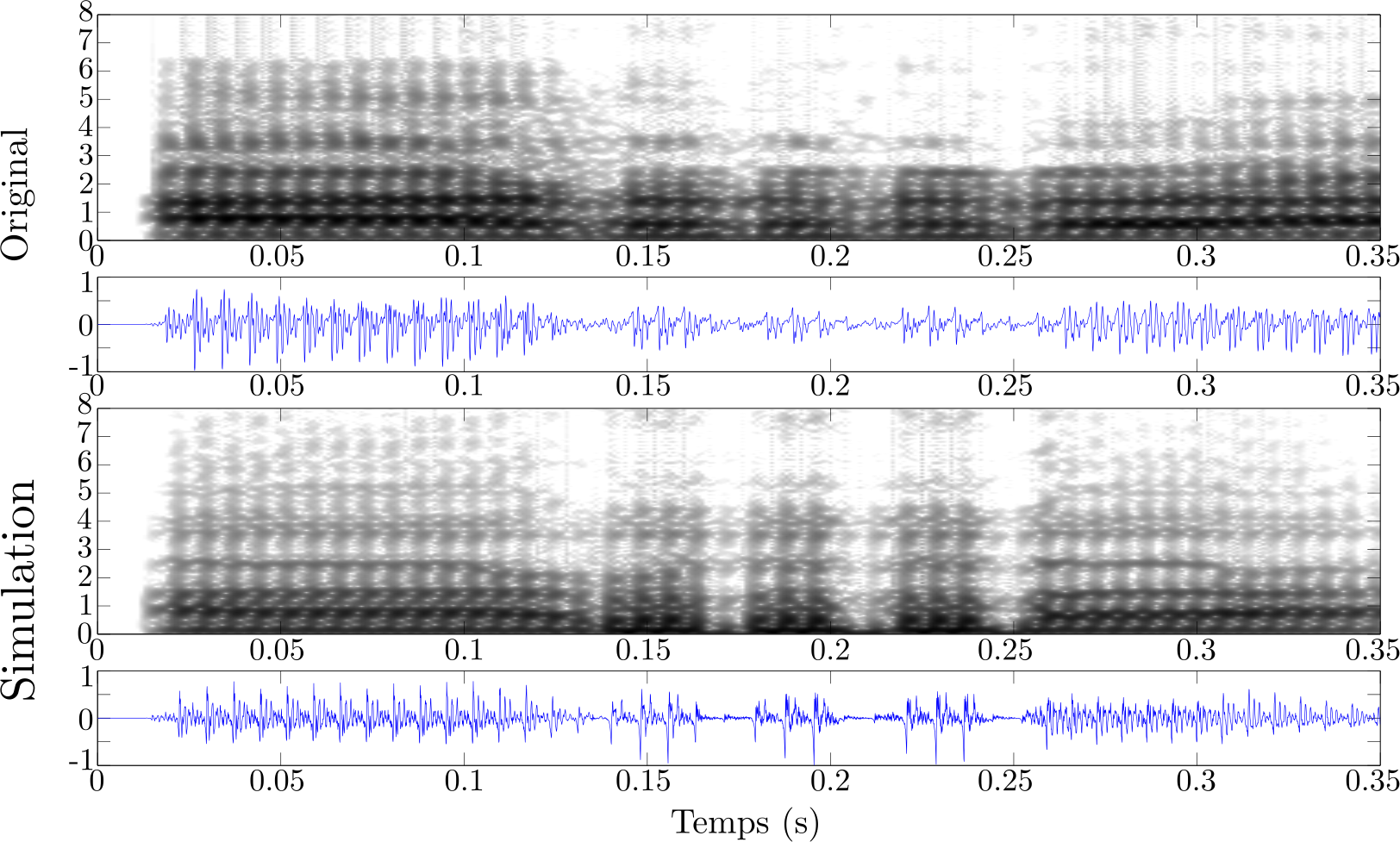
Fig. 5 - Wide-band spectrogram of the pseudoword /ara/ containing an
alveolar trill. Top is the original utterance, and bottom is the
simulated utterance. The $y$-axis represents the frequency in kHz.